Key takeaways
On this page
t is now absolutely clear that solar is taking over the world and bringing a new era of green energy generation. But its success couldn’t be solely based on environmental benefits – economic viability is a big driving factor as well. Solar power has made tremendous progress towards that end over the last decades, offering homeowners and businesses more cost-effective alternatives to fossil fuels.
As the solar research unfolded, however, it became clear that the current silicon-based technology has considerable limitations which somewhat dampen prospects for further solar deployment. Luckily, another technology has been evolving in parallel with silicon photovoltaics, which essentially led to the creation of the perovskite solar cell (PSC). It radically changes the landscape for solar power deployment and opens up new horizons for the industry.
The history of a perovskite solar cell
The history of a perovskite solar cell arcs over two centuries. It goes back to 1839 when German crystallographer Gustav Rose discovered a new metallic cube-like mineral during his expedition to the Ural Mountains in Russia. He found out it consisted of calcium and titanium oxides and had a chemical formula CaTiO3. Rose named his discovery “perovskite” as a tribute to the Russian mineralogist Lev Perovski.
The calcium titanate-based mineral still had a long way to go toward its practical application, however. It found some use in the production of condensers and electromechanical transducers in the middle of the 20th century, but true success came decades later.
CaTiO3 stepped into the spotlight only in 2009 with the creation of the perovskite solar cell based on a hybrid organic-inorganic semiconductor material. This discovery belongs to a group of scientists led by Japanese engineer Tsutomu Miyasaka. Even though the device originally showcased a fairly low performance with a solar conversion rate of only 3.8%, its promising light-absorbing capacity attracted broad attention within the scientific community.
The next big step forward in developing PSCs technology was made in 2012 when liquid electrolyte within the solar cell was replaced with a solid hole conductor. This helped to solve the problem of low thermal stability of liquid electrolytes and increased solar conversion efficiency to almost 10%. But it still wasn’t enough to challenge silicon-based PV technology.
Explore Pennsylvania solar incentives to save on installation costs & reduce energy bills. Take advantage of rebates & tax credits today!
In 2016, scientists found a way to reduce deep-seated flaws in the formation of transporting layers that negatively impacted their performance. As a result, the efficiency jumped to 22.1%. Today the PSC conversion exceeds 26%, which means it has already caught up with its silicon counterparts. And everything points to the fact that current levels could be raised even further.
Structure & working principle
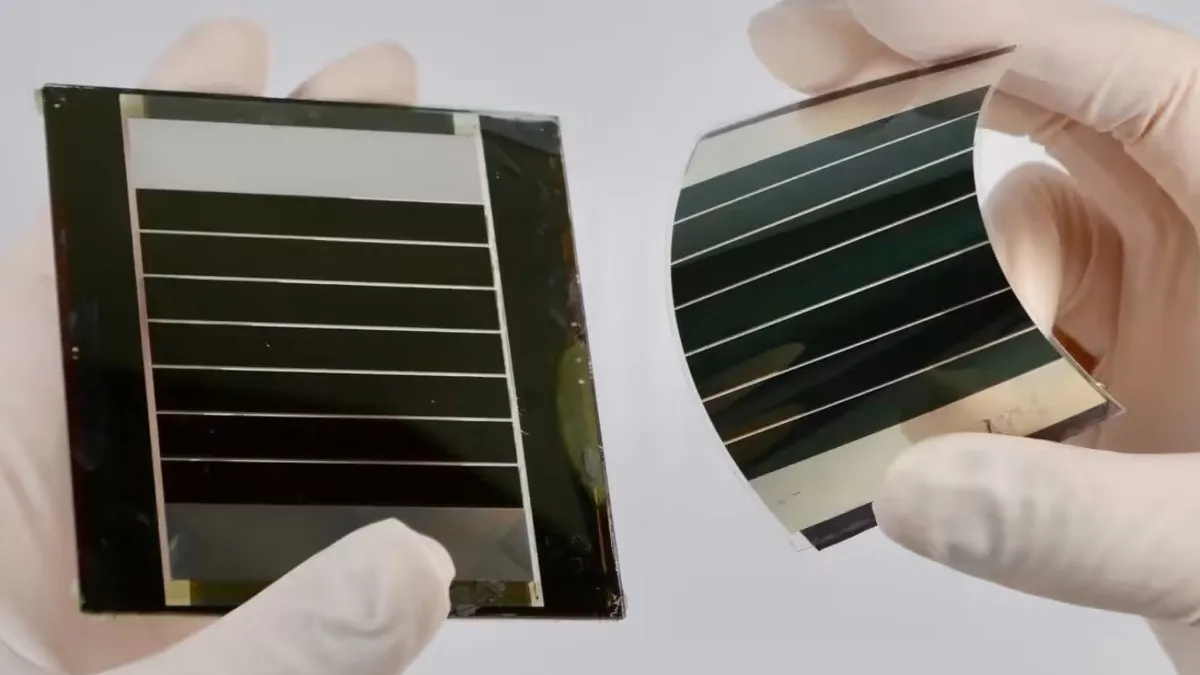
A perovskite solar cell typically has an ABX3 structure, where A is organic cation, B is inorganic cation (most commonly lead), and X is halogen anion. The solar absorption layer is sandwiched between a hole transporting layer (HTL) and an electron transporting layer (ETL). The cell has a transparent cathode on top and a metal anode at the bottom.
A perovskite solar cell can have a regular (negative-intrinsic-positive) or inverted (positive-intrinsic-negative) configuration depending on which transportation material is placed closer to the top of the cell. These types can be further broken into two categories: mesoscopic and planar. Mesoporous solar cells contain a sponge-like scaffold material while planar cells don't have it.
The working principle of a perovskite solar cell is very similar to a crystalline silicon cell - it also generates electricity using the photovoltaic effect. When solar light reaches the absorber layer, it excites electrons within it and frees them from their positions, creating empty spaces called holes. The electrons move towards the hole transporting layer, which carries them to the conductor creating an electric current. After that, the electrons are collected by the electron transporting layer.
Generally, the main difference between PSCs and crystalline silicon solar cells is that the absorbing layer in PSCs needs HTL and ETL to conduct the movement of electrons and holes effectively.
Perth Solar offers high-quality solar panel installations to harness clean energy and reduce electricity bills. Go green with Perth Solar today!
PSCs vs. silicon
Cost
Lower cost is what spurs interest in PSCs in the first place. Most solar panels available on the market today are manufactured from crystalline silicon, which requires extensive mining, purification, and treatment before it can be used to make PV panels.
The mineral used for PSCs production, on the other hand, is abundantly available in nature and much easier to process than silicon, which results in a lower final cost. In addition to this, with a new roll-to-roll coating technique PSCs can be simply printed on thin glass or plastic sheets using nanoparticle ink. This makes the production at times cheaper and opens doors to mass-scale manufacturing.
Efficiency
When it comes to performance, power conversion efficiency (PCE) is the first thing to look at. And PCE, in turn, depends on the band gap, which refers to the minimum energy required to knock the electrons loose from their parent atoms. If the gap is too high, the photons hitting the surface of the panel won’t cause photovoltaic effect. But if it is too low, the photons will provide more energy than required to set the electrons in motion, so the excess energy will be simply wasted.
The best theoretically possible direct band gap of a solar cell is 1.34 electron volts (eV), which is known as the Shockley-Queisser (S-Q) limit. This means the absorbed photons must have energy greater than 1.34 eV to move electrons from their standard positions. If a solar cell with a single positive-negative junction between semiconductor layers had an ideal bandgap it would convert about 33.7% of sunlight into electricity.
A perovskite solar cell has been slightly inferior to its silicon opponent in terms of a band gap for a long time. The room-temperature band gap of silicon modules is 1.1 eV, while PSCs typically had a band gap ranging from 1.5 eV to 1.7 eV, which was considerably higher than the maximum allowed levels of single-junction solar cells.
However, the researchers managed to optimize PSCs' solar conversion efficiency by bringing their bandgap closer to the optimum levels. This was achieved by replacing a certain amount of lead ions with tin. These mixed tin−lead PSCs have a band gap in the range of 1.2−1.3 eV, which brings them much closer to the S-Q limit and makes them even more suitable for solar PV applications than silicon-based options.
Stability
The biggest bottleneck to PSCs' commercialization is their instability, which results in a shorter lifespan - a perovskite solar cell normally loses its efficiency within a couple of days. Even though it is a huge improvement compared to early prototypes which degraded in a matter of minutes, it still isn’t enough to offer a viable alternative to conventional silicon panels.
The problem is that PSCs are highly exposed to moisture, heat, or continuous high voltages. To improve their stability, scientists are experimenting with different types of both the absorbing layer and its surrounding layers.
Those efforts are already yielding results, as new discoveries are being made every year taking us closer to massive commercialization of PSCs. According to the latest findings, a possible solution to the instability problem is changing the structure of the hole transporting material. A new state-of-the-art tin-lead PSC can keep 80% of its maximum efficiency after 1,500 hours of operation or more than 62 days.
Environmental impact
Even though conventional solar panels are undoubtedly a much greener alternative to fossil fuels, they still cause some environmental concerns. For example, silicon purification is responsible for a certain amount of CO2 emissions. Additionally, there are a lot of unresolved issues in terms of recycling and disposal of silicon-based solar modules.
A perovskite solar cell, on the other hand, has a significantly lower carbon footprint. Its manufacturing process needs much less heat and pressure and hence consumes less energy. PSCs are also easier and cheaper to recycle.
Conclusion: perovskite solar cell is a game-changing tech
Considering all the above advantages, there is no doubt that a perovskite solar cell is the future of solar energy. PSCs offer lower manufacturing costs, which means they have the potential to make solar power even more accessible. Moreover, their performance is already superior to competitors and is going to improve further, as they can be tuned to capture a wider range of wavelengths of the solar spectrum.
Another promising direction is the development of hybrid devices combining properties of both PSCs and silicon-based solar cells. Their remarkable 30% conversion efficiency and solid durability promise huge advantages for the solar industry.
Related





Key takeaways
The rise of perovskite solar cells (PSCs) is reshaping the solar energy landscape, offering a more cost-effective and efficient alternative to traditional silicon-based technology.
PSCs have a fascinating history, dating back to the discovery of the perovskite mineral in 1839. The breakthrough in PSC technology came in 2009, and subsequent advancements have boosted efficiency to over 26%, rivaling silicon counterparts.
The structure of a PSC involves organic and inorganic components, and its working principle harnesses the photovoltaic effect to generate electricity.
PSCs present a compelling cost advantage, as the minerals used are abundant and easier to process than silicon. The roll-to-roll coating technique further enables cost-effective mass production.In terms of efficiency, PSCs have narrowed the gap with silicon by optimizing the bandgap, reaching levels close to the Shockley-Queisser limit.
Stability remains a challenge, but ongoing research, including changes in the hole transporting material, shows promising results. A new tin-lead PSC maintains 80% efficiency after 1,500 hours of operation.
Environmental impact is reduced with PSCs, offering a lower carbon footprint, less energy-intensive manufacturing, and easier recycling compared to silicon-based solar panels.
PSCs emerge as a game-changing technology, with lower costs, improved efficiency, and environmental benefits, positioning them as the future of solar energy.